Crystalline Sponge Method on XtaLAB Synergy Systems
Introduction
The Crystalline Sponge (CS) method is an approach to obtain single-crystal X-ray structures of compounds, that are difficult or impossible to crystallize, for example because they are of too small a quantity, are not solid at ambient conditions or form amorphous precipitates. In some cases spectroscopic or spectrometric techniques cannot give definitive answers to the analytical problems so a crystal structure is needed.[1] The CS method utilizes a metal-organic-framework (MOF) as a host for analyte molecules (guests), see Figure 1. Analytes can diffuse into the pores of the MOF from solution or the gas-phase and align themselves in an ordered fashion, which is predetermined by binding sites of the MOF cavity. This induced-fit molecular recognition process can be understood as crystallization inside a crystal.
This method was already successfully applied in determination of bioactive compounds like terpenes[2] or steroids[3], natural products[4], metabolites[5], reactive chemical species like ozonides[6], or volatile compounds[7].
Figure 1: Comparison of conventional crystallization and the CS method. X = Cl, I
Synthesis
The crystalline sponges are rapidly prepared by carefully layering a methanol solution of zinc chloride or zinc iodide over a chloroform or nitrobenzene solution of the ligand 2,4,6-tris(4-pyridyl)- 1,3,5-triazine (tpt). At the interface of both solutions, the formation of an opaque layer is observed. From this opaque layer, crystals of size 50-100 µm can be harvested in 2-3 days, see Figure 2. A prolonged crystallization time leads to the formation of large and twinned crystals. The exchange of polar solvents from the synthesis to apolar solvents is typically done to promote the guest soaking step. Polar solvents are good guests themselves and need to be exchanged to weakly binding solvent molecules like short-chained linear alkanes, cyclohexane or trans-decalin.
Figure 2: Crystallization setup
Soaking
CS prepared in this way are subjected to the most delicate and crucial step of this method: the soaking process. Soaking describes to uptake of an analyte into the pores of the crystalline sponge cavities and typically a guest-occupancy greater than 50 % is required to resolve the analyte molecules satisfyingly. Soaking requires only up to 1 µg of substance when a sponge crystal is transferred to a conical-shaped micro-vial, submerged in 20-50 µL solvent, see Figure 3.
Figure 3: CS in micro vial
Soaking can be accelerated at elevated temperatures and by piercing a needle through the cap of the vial, allowing evaporation of the solvent and forcing the analyte molecule into the CS pores. Organic molecules with N-donor sites like amines or heterocyclic compounds may disturb the integrity of the CS framework.[8] Such compounds are often best soaked at lower temperatures (4°C) and lower analyte concentrations. Soaking conditions may need to be optimized, changing from sample to sample. Small crystalline sponges (d = 50 µm) show typically higher guest occupancy after soaking compared to larger crystals (d = 100-150 µm) which may exhibit a guest concentration gradient through the crystal pores.
Challenges of the Method and how to handle them
The synthesis of the crystalline sponges can be learned and perfected rapidly. Analytes should be purified by chromatographic methods prior to soaking, since the sponge crystals typically do not exhibit specific binding sites for all analytes. It was demonstrated that the CS method can be coupled with HPLC[1] or GC[7] allowing automation thereof. Furthermore, parameters like soaking temperature, solvent type and analyte concentration must be optimized for each analyte molecule individually to achieve good guest occupancy in the CS host framework. Overall the application of the CS method requires measurements of multiple sponge crystals, soaked with various conditions and analytes. A modern single crystal X-ray diffraction instrument with a high brilliance rotating anode X-ray source like the XtaLAB Synergy-R (Cu) or XtaLAB Synergy-DW VHF (Cu, Mo) are best suited to screen and measure multiple sponge crystals in an efficient manner, see Figure 4. XtaLAB systems might even be used in combination with an XtalCheck-S to identify the best, soaked crystals prior to full measurements.
With the CS method, one is also able to perform absolute structure determinations. Chiral guest molecules transfer their conformational information to the host framework and the space group of the CS typically changes from C2/c to C2 or even less symmetric space groups. With sufficient guest occupancy, reasonable Flack parameters are achieved.[9] Since the CS framework exhibits large amounts of zinc halide moieties, the generation of strong anomalous signal is possible with all common laboratory wavelengths.
Figure 4: XtaLAB Synergy-DW VHF equipped with a PhotonJet DW VHF source and an HyPix-Arc 150° hybrid photon counting detector (cabinet not shown for clarity)
Absorption effects from zinc halides can be easily treated with face-based absorption correction and the use of small and uniformly soaked crystalline sponges. Typically Cu-Kα is preferred over Mo-Kα because it is more intense and therefore reduces the measurement time and X-ray dose time to the crystal significantly. Complete and redundant datasets are measured in less than one hour using Cu-Kα radiation to a resolution of 0.84 Å or better, see Figure 5. The use of a curved detector with a large active area allows to collect accurate data, distortion-free, and quickly with a minimum of dose- time on the crystal.
Figure 5: Crystalline Sponge measured with a HyPix-Arc 150° detector. Diffraction is observed well beyond 0.84 Å
Furthermore accurate intensities are necessary for the strongest reflections as well as for the weakest reflections. Low noise hybrid photon-counting detectors like the HyPix-6000HE or HyPix-Arc150° should be employed, since they offer a wide dynamic range (> 106) and zero background, requiring no oversampling of frames for intensity approximations, which could lead to unnecessary sample decomposition, see figure 6.
Figure 6: Pronounced discoloration of a ZnI2 based crystalline sponge. Before measurement (left), after measurement (right)
Employing an HPC detector with 150° angular coverage allows the user to measure all reflections at one value of detector 2θ which is beneficial for scaling and acceleration of the measurement speed of sensitive crystals. Since the zinc halide moieties are strong absorbers, the data must be scaled and corrected for absorption effects to calculate accurate difference electron maps for the structure refinement of the analyte molecules inside the sponge cavities. Degradation of the sponge crystals throughout the measurement can be problematic. Our freely available, user-inspired software package CrysAlisPro provides all necessary tools for handling crystalline sponges from measurement, through scaling to structure solution and refinement using AutoChem. Refinement of B-factor encounters for the gradual degradation of the sponge framework throughout the measurement.[10]
A Crystalline Sponge measurement from start to the end
Caffeine was chosen as an appropriate guest molecule for the CS method because it is a representative example of a small molecule active pharmaceutical ingredient (API). ZnCl2 based CS crystals were prepared according to the literature described procedures.[7] A single crystal was transferred to a conical-shaped vial, along with 50 µL cyclohexane and 1 µL caffeine solution (1 µg/µL in CH2Cl2). the vial was closed with a screw cap, equipped with a PTFE liner. The liner was pierced with a needle and the solvent was allowed to evaporate at 50°C over the course of two days. Diffraction data was collected using Cu-Kα radiation on an XtaLAB Synergy-S (Cu, Mo) and a HyPix-6000HE hybrid photon counting detector at 100 K. In total 1862 frames were collected, with exposure times of 0.24 s and 4.40 s, giving a total experiment time of 1:34 hours.
Figure 7 shows the difference electron density map of the refined host framework and the modeled guest molecules of caffeine.
Figure 7: Crystal structure of caffeine inside a crystalline sponge. The guest molecules are clearly visible in the difference electron density map
Conclusion
XtaLAB Synergy systems, with their powerful rotating anodes and accurate direct photon counting technology of the HyPix detectors, are perfectly suited for fast and reliable measurements of Crystalline Sponges. Due to the optimization steps needed to perfect the soaking of guest molecules, the CS method requires ability to measure multiple crystal-sets in a short time period. It was furthermore demonstrated that that even with microfocus sealed tubes, full measurements can be achieved in reasonable time intervals.
CrysAlisPro is the freely available software of choice for data processing. Advanced scaling options allow the user to refine and obtain the best achievable data from their diffraction experiments
Further Information
A webinar on the Crystalline Sponge method is available:
https://www.rigaku.com/webinars/crystalline- sponge/method
Literature
[1] Y. Inokuma, S. Yoshioka, J. Ariyoshi, T. Arai, Y. Hitora, K. Takada, S. Matsunaga, K. Rissanen, M. Fujita, Nature 2013, 495, 461–466.
[2] T. Mitsuhashi, T. Kikuchi, S. Hoshino, M. Ozeki, T. Awakawa, S.-P. Shi, M. Fujita, I. Abe, Org. Lett. 2018, 20, 5606-5609.
[3] Y. Inokuma, T. Ukegawa, M. Hoshinoac, M. Fujita, Chem. Sci. 2016, 7, 3910–3913.
[4] S. Urban, R. Brkljaca, M. Hoshino, S. Lee, M. Fujita, Angew. Chem. Int. Ed. 2016, 55, 2678 –2682.
[5] L. Rosenberger, C. von Essen, A. Khutia, C. Kühn, K. Urbahns, K. Georgi, R. W. Hartmann, L. Badolo, Drug Metab. Dispos. 2020, accepted manuscript.
[6] S. Yoshioka, Y. Inokuma, V. Duplan, R. Dubey, M. Fujita, J. Am. Chem. Soc. 2016, 138, 10140–10142. [7] N. Zigon, T. Kikuchi, J. Ariyoshi, Y. Inokuma, M. Fujita, Chem. Asian J. 2017, 12, 1057-1061.
[8] F. Sakurai, A. Khutia, T. Kikuchi, M. Fujita, Chem. Eur. J. 2017, 23, 15035-15040.
[9] S. Lee, M. Hoshino, M. Fujita, S. Urban, Chem. Sci. 2017, 8, 1547-1550.
[10] J. Christensen, P. N. Horton, C. S. Bury, J. L. Dickerson, H. Taberman, E. F. Garmanc, S. J. Coles, IUCrJ 2019, 6, 703-713.
Related products
XtaLAB SynergyCustom
A bespoke, extremely high-flux diffractometer with custom enclosure and the flexibility to utilize both ports of the rotating anode X-ray source.
Learn MoreXtaLAB Synergy-i
An entry-level single crystal X-ray diffractometer for Chemical Crystallography configured with microfocus sealed tube technology and a direct X-ray detection detector.
Learn MoreXtaLAB Synergy-R
Our most popular diffractometer for Protein Crystallography, configured with a high-flux rotating anode X-ray source and an extremely low noise direct X-ray detection detector.
Learn MoreXtaLAB Synergy-S
Our most popular diffractometer for Chemical Crystallography and Mineralogy, configured with either single or dual microfocus sealed tube X-ray sources and an extremely low noise direct X-ray detection detector.
Learn More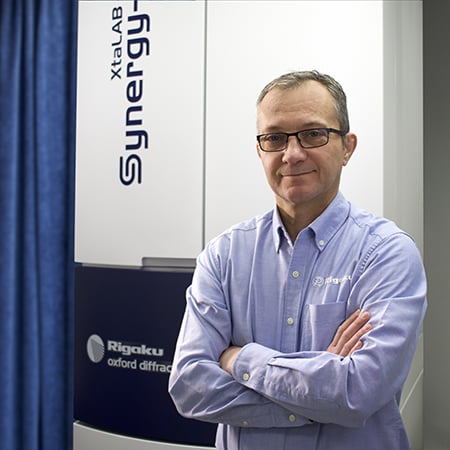
Contact Us
Whether you're interested in getting a quote, want a demo, need technical support, or simply have a question, we're here to help.