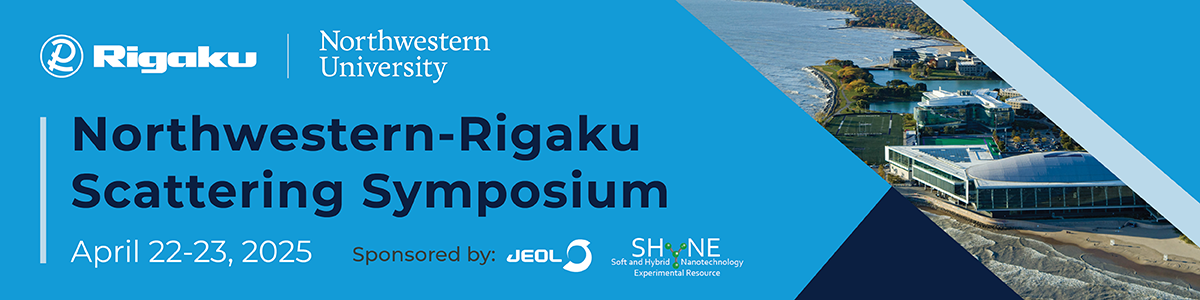
Northwestern-Rigaku Scattering Symposium
Northwestern University, in collaboration with Rigaku, is pleased to announce the Northwestern-Rigaku Symposium on April 22-23. Each morning will feature seminars from researchers at Northwestern, the University of Chicago, Colorado School of Mines, Concordia University, and Rigaku, covering the latest advancements in electron diffraction, single-crystal diffraction, XRD, and XRF. Afternoons will offer hands-on training, showcasing instrument capabilities, and software applications.
Registration is closed. Please contact Michelle Goodwin to join the standby list in case of cancellations.
Schedule at a Glance
Microcrystal electron diffraction (MicroED/3D ED), which solves atomic structures from crystals in the sub-micron size range using electron radiation, has evolved from an exploratory technique into a cutting-edge analytical method practiced in laboratories worldwide. However, viewing MicroED solely as an extension of single-crystal diffraction, traditionally performed with X-rays on smaller crystals, underestimates its capabilities. With thousands of crystallites available simultaneously on a sample grid and measurements completed within minutes, we can obtain a more comprehensive view of our specimen by collecting crystallographic data from dozens or even hundreds of crystals. This approach allows for the analysis of powders containing mixtures of compounds, different polymorphs, or even small traces of contaminants, including ab-initio solutions, even in cases too complex for powder diffraction or where only minute sample amounts are available. In this contribution, we will discuss how high-throughput workflows in the Rigaku XtaLAB Synergy-ED electron diffractometer enable various beyond-single-crystal investigations with a high degree of automation and ease of use.
Metal–organic frameworks (MOFs) are structurally diverse, porous materials comprised of metal nodes bridged by organic linkers. Through careful choice of nodes and linkers, the chemical and physical properties of MOFs can be elegantly tuned and materials with very high surface area and porosity can be obtained. As a consequence, MOFs have been explored for many potential applications including, but not limited to, gas storage and release, chemical separations, catalysis, drug delivery, light harvesting and energy conversion, and the detoxification of hazardous analytes. In the Howarth lab, we are interested in the design and synthesis of novel MOFs comprised of rare-earth (RE) ions bridged by organic linkers that can act as photosensitizers for the generation of singlet oxygen. RE ions allow for the construction of unique and intricate MOF topologies that allow for the systematic study of structure-function relationships related to singlet oxygen production and subsequent detoxification of a mustard gas simulant. In this presentation, RE-MOFs are explored for the photo-driven oxidative detoxification of a mustard gas simulant, with a focus on understanding how structural details deciphered through electron diffraction and total X-ray scattering affect photocatalytic activity.
References
- B. F. Hoskins and R. Robson, J. Am. Chem. Soc., 1989, 111, 5962; O. M. Yaghi and H. Li, J. Am. Chem. Soc., 1995, 117, 10401.
- A. J. Howarth, Y. Liu, P. Li, Z. Li, T. C. Wang, J. T. Hupp and O. K. Farha, Nat. Rev. Mater., 2016, 1, 15018.
- F. Saraci, V. Quezada-Novoa, P. R. Donnarumma, and A. J. Howarth, Chem. Soc. Rev., 2020, 49, 7949.
Within a year of the first experiments demonstrating electron diffraction, Hans Bethe solved the Schröedinger equation for dynamical diffraction,[1] demonstrating that a complete treatment was needed to explain experimental data. Quietly ignoring dynamical effects, in 1933 Laschkarew and Usyskin determined the positions of hydrogen atoms in NH₄Cl crystals.[2] In the close to a century of electron diffraction this has remained a contraversial topic. Hard-core physics says that simple, kinematical models are inadequate, whereas in practice it has often been possible to invert electron diffraction data to obtain crystal structures.
Why?
It turns out that there are quite a few cases where dynamical contributions do not destroy the possibility of inverting electron diffraction data, particularly using direct methods.[3-5] Of particular interest, averaging over angles as in precession electron diffraction or with three dimensional datasets effectively reduces dynamical contributions,[6] with good results obtained with dynamical refinements.[7] This talk will overview why electron crystallography can, and often does work without recourse to kinematical approximations.
- Bethe, H., Theory on the diffraction of electrons in crystals. Annalen Der Physik, 1928. 87(17): p. 55-129 https://doi.org/10.1002/andp.19283921704.
- Laschkarew, W.E. and I.D. Usyskin, The determination of the position of hydrogen ions in the NH(4)Cl crystal lattice by electron diffraction. Zeitschrift Fur Physik, 1933. 85(9-10): p. 618-630 https://doi.org/10.1007/Bf01331003.
- Sinkler, W., E. Bengu, and L.D. Marks, Application of direct methods to dynamical electron diffraction data for solving bulk crystal structures. Acta Crystallographica Section A, 1998. 54: p. 591-605 https://doi.org/10.1107/S0108767398001664.
- Sinkler, W. and L.D. Marks, Dynamical direct methods for everyone. Ultramicroscopy, 1999. 75(4): p. 251-268 https://doi.org/10.1016/S0304-3991(98)00062-X.
- Marks, L.D. and W. Sinkler, Sufficient conditions for Direct Methods with swift electrons. Microsc Microanal, 2003. 9(5): p. 399-410 https://doi.org/10.1017/s1431927603030332.
- Marks, L.D., Models for Precession Electron Diffraction, in Uniting Electron Crystallography and Powder Diffraction, U. Kolb, et al., Editors. 2012, Springer: Netherlands.
- Palatinus, L., et al., Structure refinement from precession electron diffraction data. Acta Crystallogr A, 2013. 69(Pt 2): p. 171-88 https://doi.org/10.1107/S010876731204946X.
Station 1: XtaLAB Synergy-ED – Overview of the hardware, sample loading, full experiment from sample screening to structure solution, introduction to high-throughput features
Station 2: XtaLAB Synergy-S and XtaLAB Synergy DW – Overview of the hardware, full experiment from sample screening to structure solution, comparison of the microfocus sealed-tube and the microfocus rotating anode.
Station 3: XRD - Overview of the hardware and a discussion about powder, thin film, micro-area, WAXS/SAXS applications. You may bring your own samples to run.
Station 4: XRF - ZSX Primus IV-Overview of operation, data collection, data processing, and elemental mapping using a tube-above 4 kW wavelength dispersive XRF instrument.
This session is open to everyone and will take place in the Willens Wing Atrium. Gift cards of $100 and $50 will be awarded to the 1st and 2nd prize winners, respectively.
Maximum dimensions for the posters: 4’ x 4’ (either in portrait or landscape format).
For information about posters, please contact Pierre Le Magueres (pierre.lemagueres@rigaku.com).
The AI-powered X-ray powder diffraction (XRPD) analysis software module enhances productivity in analysis by accurately identifying both main and minor phases, including impurities and foreign materials, without deep operator involvement. Using a neural network, it learns phase patterns from simulated data, refining its predictions through iterative training and feedback to outperform conventional search/match algorithms.
The AI-powered X-ray reflectivity (XRR) software uses a neural network to suggest adjustments to simulation models, improving accuracy. It predicts discrepancies between experimental and simulated profiles, such as missing layers, by learning from simulated data. The network refines its predictions through iterative feedback, enhancing XRR fitting precision.
Both AI-powered analysis software work great with the XSPA high-energy resolution X-ray detector that is a multidimensional photon counting pixel array detector supporting 0-D, 1-D, and 2-D measurements, enabling versatile XRPD and XRR analysis. With a high XRF background suppression, wide dynamic range, and Kβ filter-less measurement capability, it delivers precise data from diverse samples, including powders, bulk materials, and thin films.
The Rigaku SmartLab is one of the most versatile high-precision X-ray diffractometers (XRDs) for the structural characterization of materials, allowing all types of applications involving powders to thin films to surfaces. The X-Ray Diffraction Facility at the Materials Research Center of Northwestern University possesses four Rigaku SmartLab XRD instruments for multipurpose ambient as well as temperature and gas flow-controlled in situ measurements. I will present a few cases where Bedzyk’s X-ray interface science group uses the Rigaku SmartLabs to facilitate materials research in collaboration with the groups of Haile, Wolverton, Poeppelmeier, and Dravid.
Molybdenum oxynitrides are of interest as catalysts, from hydrogen evolution to oxygen reduction. Although the high temperature ammonolysis of MoO₃ precursors is commonly used to produce molybdenum oxynitride, the reaction pathway by this method remains poorly understood. We utilized in situ powder X-ray diffraction (PXRD) using a 9 kW Cu rotating anode Rigaku SmartLab equipped with an Anton Paar XRK 900 reactor chamber to comprehensively map the structural transformation from MoO₃ to HxMoO₃ to MoO₂ and finally to γ-MoOxNy.1 Our findings for the reaction pathway provide a roadmap for future tuning of the molybdenum oxynitride chemistry.
Tantalum oxynitride, specifically β-TaON, is a promising material for photocatalytic water-splitting applications. We implemented a materials discovery approach that used pulsed laser deposition (PLD) for compositionally graded TaOxNy thin-film growth and small-area XRD for phase determination.2 The Rigaku SmartLab polycapillary optic (CBO-f unit) was utilized to focus the line source beam from a 3 kW Cu X-ray tube to a 400 μm spot at the sample position and identify the β-TaON region from a library of different phases on a compositional gradient sample. This combinatorial method proves to be very efficient for the growth and discovery of a material’s phase of interest.
Hydrogen-bearing Fe-Y based perovskites provide a compelling platform for developing solid oxide fuel/electrolysis cells. One of the limiting factors for the performance of Fe-containing electrochemical cells is the chemical stability of the material under operational conditions, which needs to be studied thoroughly. We used a Rigaku SmartLab, equipped with an Anton Paar DHS1100 graphite mini chamber, for real-time high-resolution XRD studies of the structural stability of BaFe0.8Y0.2O3-δ / SrTiO₃(001) single crystal thin film under flowing 8% O₂ in a N₂ carrier gas. The high redox activity of Fe leads to a change in the crystalline structure of the film, as observed by means of lattice expansion and single crystal domain size reduction. This result provides valuable insights into the structure and chemistry of the BaFe0.8Y0.2O3-δ proton-conducting electrolyte.
References:
- Chi Zhang, Elise A. Goldfine, Kun He, Jill K. Wenderott, Shobhit A. Pandey, Roberto dos Reis, Jiahong Shen, Chris Wolverton, Michael J. Bedzyk, Kenneth R. Poeppelmeier, Vinayak P. Dravid, and Sossina M. Haile, “Elucidating the Reaction Pathway in the Ammonolysis of MoO₃ via In Situ Powder X-ray Diffraction and Transmission Electron Microscopy”, Chemistry of Materials 36 (3), 1123-1135, 2024. DOI: 10.1021/acs.chemmater.3c01344.
- K. V. L. V. Narayanachari, D. Bruce Buchholz, Elise A. Goldfine, Jill K. Wenderott, Sossina M. Haile, and Michael J. Bedzyk, “Combinatorial Approach for Single-Crystalline TaON Growth: Epitaxial β-TaON (100)/α-Al₂O₃ (012)”, ACS Applied Electronic Materials 2 (11), 3571-3576, 2020. DOI: 10.1021/acsaelm.0c00622.
The cuboidal Fe₄S₄ cluster is a ubiquitous cofactor for electron transport in biological systems. In this presentation I will describe our endeavors into artificial electrically conductive materials based on redox-active Fe₄S₄-clusters. Our initial efforts concentrated on a parent coordination polymer formed with 1,4-benzenedithiolate. The organic linker was then tuned by methylation of the dithiolate ligand to obtain a series of new Fe₄S₄-based coordination polymers (2,5-dimethyl-1,4-benzenedithiol and 2,3,5,6-tetramethyl-1,4-benzenedithiol). A subsequent screen over a variety of cations also rendered variations of amorphous and polycrystalline materials. Growing large single crystals suitable for single crystal X-ray diffraction proved to be problematic in this system.
In this presentation, I will concentrate on the development of structural models of solid materials based on synchrotron powder diffraction data (via simulated annealing structure solution followed by a Rietveld refinement) and most recently using electron diffraction data collected on Rigaku XtaLAB Synergy-ED instrument. Determination of several crystal structures based on different thiolates and cations were a culmination of our efforts.
Station 1: XtaLAB Synergy-ED – Overview of the hardware, sample loading, full experiment from sample screening to structure solution, introduction to high-throughput features
Station 2: XtaLAB Synergy-S and XtaLAB Synergy DW – Overview of the hardware, full experiment from sample screening to structure solution, comparison of the microfocus sealed-tube and the microfocus rotating anode.
Station 3: XRD - Overview of the hardware and a discussion about powder, thin film, micro-area, WAXS/SAXS applications. You may bring your own samples to run.
Station 4: XRF - ZSX Primus IV-Overview of operation, data collection, data processing, and elemental mapping using a tube-above 4 kW wavelength dispersive XRF instrument.
Contact: Michelle Goodwin
Event coordinator
Michelle.Goodwin@rigaku.com