Total Scattering Measurement
Measurement for PDF analysis
The measurement for PDF analysis is called a Total Scattering Measurement because it uses not only diffraction peaks but also broad scattering intensity arising from the local structure (diffuse scattering), which is treated as background in powder XRD measurement, for analysis.
Data from total scattering measurements must be devised in such a way that extra scattering intensity from the instrument (parasitic scattering) is not included.
Total scattering intensity is only required the information from the sample and container (or air scattering intensity) but is best to exclude any of the following components: parasitic scattering from the instrument, the background contamination due to X-ray fluorescence, etc.
In addition, there are three points to be considered for data acquisition.
1) X-ray wavelengths
The scattering vector is defined according to the following equation, which means that the shorter incident X-ray wavelengths (i.e., higher-energy X-rays) can measure the total scattering data with the widest $Q$ -range.
\begin{equation} Q = \frac{4\pi}{\lambda} \sin \theta \nonumber \end{equation}
In laboratories, Mo Kα and Ag Kα raditions are often used for the total scattering measurement.
SiO₂ glass samples measured with Cu Kα, Mo Kα, and Ag Kα radiations are shown with 2θ on the horizontal axis (left figure) and $Q$ on the horizontal axis (right figure). The three profiles feature the same measurement 2θ angles. Mo Kα and Ag Kα radiations can measure total scattering intensity with a wider Q range than that of Cu Kα radiation.
Figure 4: Profiles of SiO₂ glass measured at various wavelengths (left: horizontal axis θ right: horizontal axis Q)
So, what is the benefit of measuring total scattering intensity with a wide $Q$ range?
$G(r)$ obtained from the Fourier transform of $S(Q)$ has a finite peak width that is unrelated to the structural disorder, caused by a termination error arising from the measurable maximum $Q$ value $Q_{\max}$ and it can be easily estimated using the direct-resolution $\Delta r$ defined as follows:
\begin{equation} \Delta r = \frac{\pi}{Q_{\max}} \nonumber \end{equation}
In the case of X-rays, the condition $2\theta=180^{\circ}$ can be used to estimate a convenient $\Delta r$, which is ¼ of the wavelength of the X-rays used in the measurement.
\begin{equation} \Delta r \approx \frac{\lambda}{4} \nonumber \end{equation}
Figure. 4 shows the RDF of SiO₂ glass for each X-ray wavelength.
Si-O correlations are observed at all wavelengths. Although the RDFs of Mo Kα and Ag Kα radiations can detect the two distinct peaks related to O-O and Si-Si correlation, with Cu Kα radiation the two peaks are originally observed as a single peak because the real-spatial resolution is lower (i.e., larger $\Delta r$ value). These results indicate that the shorter-wavelength Mo Kα and Ag Kα radiations are recommended for laboratory equipment.
Figure 5: Radial distribution function $R(r)$ measured at each wavelength
Next, we would like to consider which wavelength should be selected, Mo Kα or Ag Kα radiation.
As mentioned previously, one might think that the Ag Kα line, with its higher real-space resolution Δr, would be better for total scattering measurements. However, depending on the sample to be measured, X-ray fluorescence from the sample may occur, making analysis difficult. Figure 6 shows the compatible wavelengths for the elements of note.
Figure 6: Wavelengths of X-rays compatible with each element
Figure 7 shows the total scattering profiles of ZrO₂ measured using Mo Kα and Ag Kα radiation. Although the total scattering profiles using Mo Kα radiation shows high S/N with a low background, that using Ag Kα radiation shows low S/N (shows small diffracted peaks) due to the background contamination of X-ray fluorescence.
Figure 7: Total scattering profiles of ZrO₂ measured with Ag Kα (blue) Mo Kα (red)
Therefore, Mo Kα radiation is the appropriate X-ray source for total scattering measurements if samples contain a small amount of ZrO₂, which is often used as a material for ball milling. To reduce the background contamination from X-ray fluorescence, the narrow detector's discriminator window cannot fully perform a total scattering measurement because the detector setting only partially measures Compton scattering.
2) Measurement method (reflection or transmission) and absorption correction
There are two measurement methods: transmission (e.g., Debye Scherrer geometry) and reflection (e.g., Bragg-Brentano geometry). The transmission method is often used, and the sample is often filled into a capillary. In such cases, the empty capillary profile is used as background data and must be subtracted from the data for the sample sealed in the capillary. In the case of the reflection method using Mo Kα or Ag Kα, be careful not to observe scattering from the sample plate due to the deeper penetration depth of the sample. In addition, absorption of X-rays by the sample cannot be avoided in either method. The PDF plug in supports absorption correction in cylindrical transmission, symmetric plate transmission, and symmetric plate reflection (Figure 8).
Figure 8: Schematic diagram of the measurement method
3) Measurement condition (Intensity)
Among the measurement conditions that can be set by the user, the parameters that vary in intensity are the scanning speed and sampling interval. The figures below show 2θ profiles and PDF profiles of SiO₂ glass with varying intensity at different scanning speeds. The intensity of the peak tops is shown in the left figure, and the PDF on the right corresponding to that color shows that the lower the intensity, the more noise has been included. Therefore, it is necessary to devise ways to obtain high intensity, and instrument-dependent parameters such as the X-ray source and detector are also important.
Figure 9: Measured 2θ profile (left) and PDF profile (right)
Introduction of SmartLab
We would like to introduce the features of SmartLab (goniometer) as an instrument for total scattering measurement. The goniometer is equipped with an X-ray source, a multi-layer mirror for monochromatizating incident X-rays, a sample stage, and a detector, excluding the slits.
Goniometer
The measurement angle can operate up to $160° in 2θ with the incident angle fixed at 0° (see figure below). SmartLab provides high angular resolution because the goniometer radius is 300 mm.
Figure 10: Inside of SmartLab (top: 2θ=160° placement, capillary installation bottom, movie)
X-ray source
Sealed tube and rotating cathode tube can be selected, and their respective outputs are summarized in the table below. Rotating cathode fluorescent tubes are the highest-output tubes among laboratory equipment.
Table: Output of each target
Wavelength | Output(kW) (Sealed tube) |
Output(kW) (Rotating anode tube) |
Mo Kα | 3.0 kW (60 kV-50 mA) |
9.0 kW (60 kV-150 mA) |
Ag Kα | 2.16 kW (60 kV-36 mA) |
6.0 kW (60 kV-100 mA) |
Multilayer mirror
The use of a CBO-E with multilayer mirrors makes it possible to focus the X-ray beam onto the detector, making it possible to acquire high-intensity, high-resolution data using the transmission method.
Sample stage
A capillary attachment for the transmission method allows easy mounting of the sample. Figure. 10 shows the procedure for mounting the capillary on the attachment as a movie. You can easily set up the sample for total scattering measurement.
Detector
The total scattering measurement requires a detector with high-efficiency detection for high-energy X-rays. Therefore, Rigaku offers D/teX Ultra250 HE and HyPix-3000 HE equipped with a thicker Si sensor compared with the detectors for Cu Kα radiation. Although detectors equipped with CdTe sensors are often recommended for PDF analysis, note that, for example, the absorption rate of Mo Kα and Ag Kα at high-energy wavelengths is 100%, but the detection efficiency of X-rays is not 100%, and the detection efficiency at low-energy wavelengths, such as Cu Kα, is significantly reduced.
Data comparison between SmartLab and Synchrotron
The structure factor $S(Q)$ measured with a SmartLab and at a synchrotron radiation facility (SPring-8, BL04B2) are shown in Figure 11. Good agreement between the two shows that the total scattering pattern measured with SmartLab is comparable to that at a synchrotron. In addition, if you purchase the PDF analysis option, we calibrate the instrument status for the total scattering measurement for each customer. Therefore, you can measure and analyze without troublesome settings.
Figure 11: Comparison of structure factor $S(Q)$ of SiO₂ glass measured with SmartLab and at BL04B2 (SPring-8)
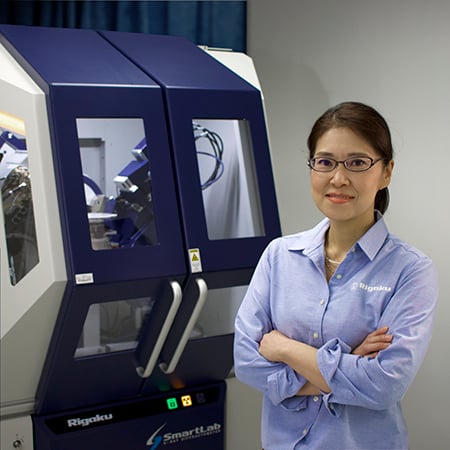
Contact Us
Whether you're interested in getting a quote, want a demo, need technical support, or simply have a question, we're here to help.