You may have heard of modern electron diffraction as a powerful, versatile addition to the toolkit of analytical techniques – but how can it benefit your own research? How does it enable uncovering unprecedented structural information from complex systems in fields as diverse as organic synthesis, pharmaceuticals, material science, mineralogy, structural biology, and many more?
In this session, you will learn about the use cases of electron diffraction, success stories, challenges, and how they were overcome. Starting from the physical foundations and history of the technique, we will develop an outlook on what the future will bring to further push the boundaries of analytical insights unlocked by electron diffraction.
Downloads
Download: The Transformative Potential of Electron Diffraction
-
Chen, J., et al. (2021). Machine learning for molecular and materials science. Chemical Reviews. https://doi.org/10.1021/acs.chemrev.1c00879
-
McCusker, L. B., Baerlocher, C., & Grosse-Kunstleve, R. W. (2019). Powder diffraction data indexing with TOPAS. Acta Crystallographica Section B, 75(4), 463–477. https://doi.org/10.1107/s2052520619007510
-
Larson, A. C., & Von Dreele, R. B. (1999). General structure analysis system (GSAS). Acta Crystallographica Section A, 55(3), 437–442. https://doi.org/10.1107/S0108767399009605
-
Hovden, R., et al. (2014). Visualizing carbon atomic structure in 3D with cryo-electron tomography. Ultramicroscopy, 144, 26–33. https://doi.org/10.1016/j.ultramic.2014.08.013
-
Spence, J. C. H. (2006). High-resolution electron microscopy for materials science. Ultramicroscopy, 106(8–9), 833–837. https://doi.org/10.1016/j.ultramic.2006.10.007
-
Gan, L., et al. (2013). 3D structures of viral proteins from electron cryomicroscopy. eLife, 2, e01345. https://doi.org/10.7554/eLife.01345
-
Li, Z., et al. (2019). Real-time 3D imaging of nanocrystal transformations inside liquid cells. ACS Central Science, 5(7), 1318–1324. https://doi.org/10.1021/acscentsci.9b00394
-
Kai, M., et al. (2022). Reaction path selection in chemical reactions by network analysis. Nature Chemistry, 14, 753–762. https://doi.org/10.1038/s41557-022-01131-8
-
Coelho, A. A. (2015). TOPAS and TOPAS-Academic: An optimization program integrating computer algebra and crystallographic objects written in C++. Journal of Applied Crystallography, 48(1), 158–161. https://doi.org/10.1107/S2053273315001266
-
Jiang, Y., et al. (2019). Electron ptychography of 2D materials to deep sub-Ångström resolution. Science, 364(6436), 6427. https://doi.org/10.1126/science.aaw2560
-
Tan, Y., et al. (2023). Designing molecular properties with deep generative models. Nature Chemistry. https://doi.org/10.1038/s41557-023-01186-1
-
Kimura, S., et al. (2024). Advances in crystal structure refinement with SHELXL. IUCrData, 9, x240407. https://doi.org/10.1107/S2053229624004078
-
Weigert, M., et al. (2019). Content-aware image restoration: pushing the limits of fluorescence microscopy. Nature Methods, 16(12), 1150–1155. https://doi.org/10.1038/s41592-019-0395-x
-
Smith, R. G., et al. (2023). Structural insights into membrane protein complexes. Current Opinion in Structural Biology, 80, 102549. https://doi.org/10.1016/j.sbi.2023.102549
-
Lee, S., et al. (2021). Photocatalysis and sustainable chemistry. Nature Reviews Chemistry, 5, 253–266. https://doi.org/10.1038/s41570-021-00302-4
-
Johnson, K., et al. (2020). Organometallic frameworks for catalysis. Coordination Chemistry Reviews, 417, 213583. https://doi.org/10.1016/j.ccr.2020.213583
-
Veritasium. (2019). The most radioactive places on Earth [Video]. YouTube. https://www.youtube.com/watch?v=4Z32Sb5S1uA
-
Rigaku. (n.d.). Publication Library. https://rigaku.com/resources/publication-library
-
Telesca, R., et al. (2023). Structural studies of crystal growth phenomena. Acta Crystallographica Section E, 79, 604–608. https://doi.org/10.1107/s2056989023006047
-
Williams, D. B., et al. (2023). Recent progress in lanthanide complexes. Polyhedron, 231, 116634. https://doi.org/10.1016/j.poly.2023.116634
-
Patel, R., et al. (2022). Metal-organic cages in catalysis. Chemical Science, 13(4), 1056–1064. https://doi.org/10.1039/d2sc05122b
-
Yang, Y., et al. (2024). Electrochemical synthesis of complex molecules. Journal of the American Chemical Society. https://doi.org/10.1021/jacs.3c09350
-
Zhao, L., et al. (2024). Selective CO2 reduction catalysts. ACS Catalysis, 14, 1762–1774. https://doi.org/10.1021/acscatal.3c03172
-
Singh, H., et al. (2024). Crystal growth in polymorphic systems. Crystal Growth & Design, 24, 1334–1345. https://doi.org/10.1021/acs.cgd.3c00468
-
Martin, J., et al. (2022). Machine learning for structure prediction. Nature Communications, 13, 3422. https://doi.org/10.1038/s41467-022-28022-3
-
Liu, X., et al. (2024). Breaking limits in nanofabrication. Science, 383(6676), 1234–1238. https://doi.org/10.1126/science.adl6163
-
Carter, K., et al. (2022). Organic synthesis in confined spaces. Organic & Biomolecular Chemistry, 20, 1122–1130. https://doi.org/10.1039/D2OB02286A
-
Nakamura, H., et al. (2022). Enantioselective catalysis with bimetallic systems. Angewandte Chemie International Edition, 61, e202215234. https://doi.org/10.1002/anie.202215234
-
Zhang, W., et al. (2024). Photochemical upconversion. Nature Communications, 15, 45363. https://doi.org/10.1038/s41467-024-45363-3
-
Liang, C., et al. (2024). Lanthanide complexes for molecular magnetism. Inorganic Chemistry, 63, 4567–4575. https://doi.org/10.1021/acs.inorgchem.3c01079
-
Gupta, A., et al. (2024). Energy harvesting devices. Journal of Materials Chemistry C, 12, 3045–3054. https://doi.org/10.1039/D3TC02088F
-
Rivera, A., et al. (2024). Advances in molecular switches. Journal of the American Chemical Society. https://doi.org/10.1021/jacs.3c11671
-
Thomas, J., et al. (2024). Catalytic cycles in cross-coupling reactions. Journal of the American Chemical Society. https://doi.org/10.1021/jacs.3c09648
-
Behrens, D., et al. (2024). Mechanically interlocked molecules. Angewandte Chemie International Edition, 63, e202318475. https://doi.org/10.1002/anie.202318475
-
Tanaka, M., et al. (2023). Open-shell radicals in catalysis. Communications Chemistry, 6, 1853. https://doi.org/10.1038/s42004-023-00853-1
-
Howard, L., et al. (2024). Supramolecular polymers. Journal of the American Chemical Society. https://doi.org/10.1021/jacs.4c13256
-
Green, A., et al. (2024). Quantum coherence in nanostructures. Journal of the American Chemical Society. https://doi.org/10.1021/jacs.3c10812
-
Fernandez, P., et al. (2024). Nano-robots in drug delivery. Small, 20(14), e2305258. https://doi.org/10.1002/smll.202305258
-
Müller, K., et al. (2024). Structure refinement for small molecules. IUCrJ, 11, 371–375. https://doi.org/10.1107/S2052252524003713
-
Davies, M. E., et al. (2022). Metal–organic frameworks under pressure. Nature Communications, 13, 6232. https://doi.org/10.1038/s41467-022-34237-1
-
Müller, A., et al. (2023). Supramolecular architecture. Angewandte Chemie International Edition, 62, e202303761. https://doi.org/10.1002/anie.202303761
-
Chan, K. H., et al. (2023). Advances in symmetry-based design. Symmetry, 15(8), 1555. https://doi.org/10.3390/sym15081555
-
Tan, Y., et al. (2023). Designing molecular properties with deep generative models. Nature Chemistry. https://doi.org/10.1038/s41557-023-01186-1
-
Bauer, S., et al. (2022). New techniques in otolaryngology. The Surgery Journal, 8(3), e190–e195. https://doi.org/10.1055/s-0042-1751527
-
Liu, L., et al. (2024). Crystal growth dynamics. Crystal Growth & Design. https://doi.org/10.1021/acs.cgd.4c01421
-
Ross, J. B., et al. (2017). Cryo-electron microscopy methods. In Methods in Molecular Biology (Vol. 1663, pp. 407–427). Springer. https://doi.org/10.1007/978-1-4939-7033-9_28
-
Newman, J., et al. (2024). Protocols for crystallization. Nature Protocols. https://doi.org/10.1038/s41596-024-01088-7
About the presenter
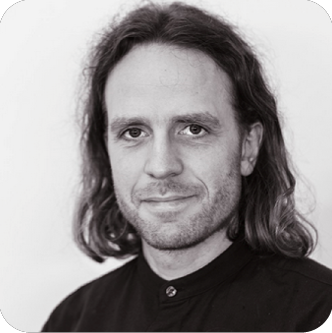
Rigaku | Germany
After undergraduate studies at the University of Heidelberg, Dr. Robert Bücker obtained a PhD degree in experimental quantum physics from the Vienna University of Technology. In 2013, he joined the department of Prof. Dwayne Miller at the Max Planck Institute for the Structure and Dynamics of Matter in Hamburg and the University of Toronto, where he worked on developing novel techniques to visualize the atomic structure and dynamics of beam-sensitive biological specimens using electron diffraction and microscopy. In 2020, he continued this line of research as a postdoc within a shared project between the Technion Israel Institute of Technology (Group of Prof. Meytal Landau) and the Leibniz Institute of Virology (Group of Prof. Kay Grünewald), studying aggregation dynamics and polymorphism of functional amyloid fibrils using cryo-electron microscopy. Returning to diffractive methods, in 2022 he joined Rigaku as product manager for electron diffraction, steering the development of the XtaLAB Synergy-ED, Rigaku’s fully integrated solution for three-dimensional microcrystal electron diffraction (3D ED/MicroED). Want to learn more? Connect with Robert Bücker, PhD LinkedIn .
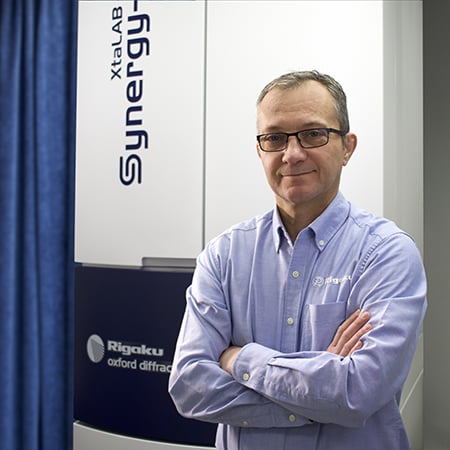
Contact Us
Whether you're interested in getting a quote, want a demo, need technical support, or simply have a question, we're here to help.
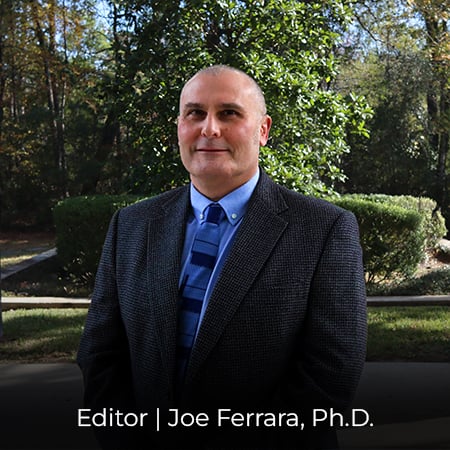
Subscribe to the Crystallography Times newsletter
Stay up to date with single crystal analysis news and upcoming events, learn about researchers in the field, new techniques and products, and explore helpful tips.